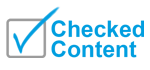
Isotope
Did you know...
SOS Children offer a complete download of this selection for schools for use on schools intranets. Sponsor a child to make a real difference.
Isotopes are any of the different forms of an element each having different atomic mass ( mass number). Isotopes of an element have nuclei with the same number of protons (the same atomic number) but different numbers of neutrons. Therefore, isotopes have different mass numbers, which give the total number of nucleons—the number of protons plus neutrons.
A nuclide is any particular atomic nucleus with a specific atomic number Z and mass number A; it is equivalently an atomic nucleus with a specific number of protons and neutrons. Collectively, all the isotopes of all the elements form the set of nuclides. The distinction between the terms isotope and nuclide has somewhat blurred, and they are often used interchangeably. Isotope is best used when referring to several different nuclides of the same element; nuclide is more generic and is used when referencing only one nucleus or several nuclei of different elements. For example, it is more correct to say that an element such as fluorine consists of one stable nuclide rather than that it has one stable isotope.
In IUPAC nomenclature, isotopes and nuclides are specified by the name of the particular element, implicitly giving the atomic number, followed by a hyphen and the mass number (e.g. helium-3, carbon-12, carbon-13, iodine-131 and uranium-238). In symbolic form, the number of nucleons is denoted as a superscripted prefix to the chemical symbol (e.g. 3He, 12C, 13C, 131I and 238U).
About 339 nuclides occur naturally on Earth, of which about 79% are stable. Counting the radioactive nuclides not found in nature that have been created artificially, more than 3100 nuclides are currently known.
History of the term
The term isotope was coined in 1913 by Margaret Todd, a Scottish doctor, during a conversation with Frederick Soddy (to whom she was distantly related by marriage). Soddy, a chemist at Glasgow University, explained that it appeared from his investigations as if several elements occupied each position in the periodic table. Hence Todd suggested the Greek term for "at the same place" as a suitable name. Soddy adopted the term and went on to win the Nobel Prize for Chemistry in 1921 for his work on radioactive substances.
In 1913, as part of his exploration into the composition of canal rays, JJ Thomson channeled a stream of ionized neon through a magnetic and an electric field and measured its deflection by placing a photographic plate in its path. Thomson observed two patches of light on the photographic plate (see image on right), which suggested two different parabolas of deflection. Thomson concluded that some of the atoms in the gas were of higher mass than the rest.
Variation in properties between isotopes
Chemical and atomic properties
A neutral atom has the same number of electrons as protons. Thus, different isotopes of a given element all have the same number of protons and electrons and the same electronic structure, and because the chemical behavior of an atom is largely determined by its electronic structure, different isotopes exhibit nearly identical chemical behaviour. The main exception to this is the kinetic isotope effect: due to their larger masses, heavier isotopes tend to react somewhat more slowly than lighter isotopes of the same element. This is most pronounced for protium (1H) vis-à-vis deuterium (2H), because deuterium has twice the mass of protium. The mass effect between deuterium and the relatively light protium also affects the behavior of their respective chemical bonds, by means of changing the centre of gravity ( reduced mass) of the atomic systems. However, for heavier elements, the absolute mass of nucleus relative to electrons if far more, and the relative mass difference between isotopes is much less, and thus the mass-difference effects on chemistry are usually negligible.
Similarly, two molecules which differ only in the isotopic nature of their atoms ( isotopologues) will have identical electronic structure and therefore almost indistinguishable physical and chemical properties (again with deuterium providing the primary exception to this rule). The vibrational modes of a molecule are determined by its shape and by the masses of its constituent atoms. Consequently, isotopologues will have different sets of vibrational modes. Since vibrational modes allow a molecule to absorb photons of corresponding energies, isotopologues have different optical properties in the infrared range.
Nuclear properties and stability
Atomic nuclei consist of protons and neutrons bound together by the strong nuclear force. Because protons are positively charged, they repel each other. Neutrons, which are electrically neutral, allow some separation between the positively charged protons, reducing the electrostatic repulsion. Neutrons also stabilize the nucleus because at short ranges they attract each other and protons equally by the strong nuclear force, and this extra binding force also offsets the electrical repulsion between protons. For this reason, one or more neutrons are necessary for two or more protons to be bound into a nucleus. As the number of protons increases, an increasing ratio of neutrons are needed to form a stable nucleus (see graph at right). For example, although the neutron:proton ratio of 3He is 1:2, the neutron:proton ratio of 238U is greater than 3:2. As a rule, there is, for each atomic number (each element) only a handful of stable isotopes, the average being 3.4 stable isotopes per element which has any stable isotopes. Sixteen elements have only a single stable isotope, while the largest number of stable isotopes observed for any element, is ten.
Although isotopes exhibit nearly identical electronic and chemical behavior, their nuclear behaviour varies dramatically. Adding neutrons to isotopes can vary their nuclear spins and nuclear shapes, causing differences in neutron capture cross-sections and gamma spectroscopy and nuclear magnetic resonance properties.
If too many or too few neutrons are present with regard to the optimum ratio, the nucleus becomes unstable and subject to certain types of nuclear decay. Unstable isotopes with a non-optimal number of neutrons decay by alpha decay, beta decay, or other exotic means, such as spontaneous fission and cluster decay.
Occurrence in nature
Elements are composed of one or more naturally occurring isotopes, which are normally stable. Some elements have unstable (radioactive) isotopes, either because their decay is so slow that a fraction still remains since they were created (examples: uranium, potassium), or because they are continually created through cosmic radiation (tritium, carbon-14) or by decay from an isotope in the first category (radium, radon).
Only 80 elements have stable isotopes, and 16 of these have only one stable isotope. Most elements occur naturally on Earth in multiple stable isotopes, with the largest number of stable isotopes for an element being ten, for tin (element number 50). There are about 94 elements found naturally on Earth (up to plutonium, element 94, inclusive), though some are detected only in very tiny amounts, such as plutonium-244. Lindsay estimates that the elements which occur naturally on Earth (some only as radioisotopes) occur as 339 isotopes (nuclides) in total. Only 269 of these naturally occurring isotopes are stable, and the other 70 are radioactive, but occur on Earth due to their relatively long half-lives, or from other means of natural production. An additional ~ 2700 radioactive isotopes not found in nature have been created in nuclear reactors and in particle accelerators. Many short-lived isotopes not found naturally on Earth have also been observed by spectroscopic analysis, being naturally created in stars or supernovae. An example is aluminium-26, which is not naturally found on Earth, but which is found in abundance on an astronomical scale.
The tabulated atomic masses of elements are averages that account for the presence of multiple isotopes with different masses. A good example is chlorine, having the composition 35Cl, 75.8%, and 37Cl, 24.2%, giving an atomic mass of 35.5. Values like this confounded scientists before the discovery of isotopes, as most light element atomic masses are close to integer multiples of hydrogen.
According to generally accepted cosmology only the isotopes of hydrogen and helium, and traces of some isotopes of lithium, beryllium and boron were created at the big bang, while all others were synthesized in stars and supernovae. (See nucleosynthesis.) Their respective abundances on Earth result from the quantities formed by these processes, their spread through the galaxy, and their rates of decay. After the initial coalescence of the solar system, isotopes were redistributed according to mass, and the isotopic composition of elements varies slightly from planet to planet. This sometimes makes it possible to trace the origin of meteorites.
Molecular mass of isotopes
The molecular mass (Mr) of an element is determined by its nucleons. For example, Carbon-12 (12C) has 6 Protons and 6 Neutrons. When a sample contains two isotopes the equation below is applied where Mr(1) and Mr(2) are the molecular masses of each individual isotope, and % abundance is the percentage abundance of that isotope in the sample.
Applications of isotopes
Several applications exist that capitalize on properties of the various isotopes of a given element.
Use of chemical and biological properties
- Isotope analysis is the determination of isotopic signature, the relative abundances of isotopes of a given element in a particular sample. For biogenic substances in particular, significant variations of isotopes of C, N and O can occur. Analysis of such variations has a wide range of applications, such as the detection of adulteration of food products. The identification of certain meteorites as having originated on Mars is based in part upon the isotopic signature of trace gases contained in them.
- Another common application is isotopic labeling, the use of unusual isotopes as tracers or markers in chemical reactions. Normally, atoms of a given element are indistinguishable from each other. However, by using isotopes of different masses, they can be distinguished by mass spectrometry or infrared spectroscopy (see "Properties"). For example, in 'stable isotope labeling with amino acids in cell culture ( SILAC)' stable isotopes are used to quantify proteins. If radioactive isotopes are used, they can be detected by the radiation they emit (this is called radioisotopic labeling).
- A technique similar to radioisotopic labelling is radiometric dating: using the known half-life of an unstable element, one can calculate the amount of time that has elapsed since a known level of isotope existed. The most widely known example is radiocarbon dating used to determine the age of carbonaceous materials.
- Isotopic substitution can be used to determine the mechanism of a reaction via the kinetic isotope effect.
Use of nuclear properties
- Several forms of spectroscopy rely on the unique nuclear properties of specific isotopes. For example, nuclear magnetic resonance (NMR) spectroscopy can be used only for isotopes with a nonzero nuclear spin. The most common isotopes used with NMR spectroscopy are 1H, 2D,15N, 13C, and 31P.
- Mössbauer spectroscopy also relies on the nuclear transitions of specific isotopes, such as 57Fe.
- Radionuclides also have important uses. Nuclear power and nuclear weapons development require relatively large quantities of specific isotopes. The process of isotope separation represents a significant technological challenge, but more so with heavy elements such as uranium or plutonium, than with lighter elements such as hydrogen, lithium, carbon, nitrogen, and oxygen. The lighter elements are commonly separated by gas diffusion of their compounds such as CO and NO. Uranium isotopes have been separated in bulk by gas diffusion, gas centrifugation, laser ionization separation, and (in the Manhattan Project) by a type of production mass spectroscopy.